Complex physical forces are at play whenever we walk, lift a cup of coffee, or swing a tennis racket. From everyday actions to elite sports performance, movement is a vital part of life. But have you ever wondered what governs these movements? This is where biomechanics comes into play—a fascinating branch of science that explores how living beings move and respond to physical forces.
In this blog, we will explore biomechanics, its various types, its fundamental principles, and its real-world applications. Whether you're a student, athlete, physiotherapist, engineer, or simply curious, this comprehensive guide will give you a solid understanding of this crucial field.
What is Biomechanics?
Let’s begin by answering a fundamental question: What is biomechanics?
At its core, biomechanics is the scientific study of the mechanical laws relating to the movement or structure of living organisms. This multidisciplinary science sits at the crossroads of biology, physics, engineering, and physiology.
Biomechanics defines how forces interact with the human body, from muscles and bones to ligaments and joints. The biomechanical meaning stems from applying principles of physics and engineering to the human body.
The term is derived from Greek roots: bio (life) and mechanics (study of motion and forces). When experts define biomechanics, they often describe it as the bridge between biology and mechanical principles. It's known as biomecanica in Spanish, and professionals in the field are called biomechanists.
Historical Background
The definition of biomechanical science has evolved through the centuries. Aristotle studied animal motion as early as 300 B.C., proposing early ideas about muscle activity and joint mechanics. Later, Leonardo da Vinci used mechanical principles to analyze walking and muscle function.
Modern biomechanics emerged in the 20th century as tools from engineering and physics became integrated into biological studies. Today, with technological advancements like motion capture and 3D modeling, the field is more dynamic than ever.
Key Objectives of Biomechanics
The goals of biomechanical analysis are multifaceted:
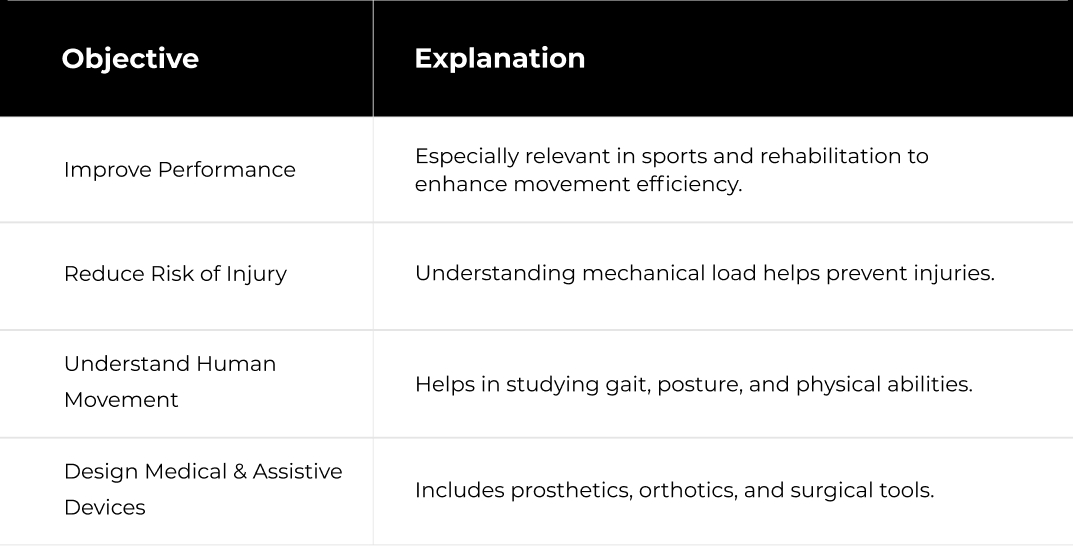
Types of Biomechanics
The scope of biomech is vast, covering various domains of biological systems. Below are the key categories:
1. Human Biomechanics
This is the most well-known form. Human biomechanics focuses on how the human body moves and functions, particularly the musculoskeletal system. It plays a pivotal role in sports science, physical therapy, orthopedics, and ergonomics.
- Example: A biomechanist may analyze an athlete’s sprinting technique to increase speed and minimize injury.
2. Animal Biomechanics
This branch looks at how animals move. From the aerodynamics of bird flight to the swimming motion of dolphins, biomechanics helps understand these unique adaptations. It also has implications in robotics and evolutionary biology.
- Example: Engineers study cheetahs' limb mechanics to design faster robots.
3. Plant Biomechanics
Yes, even plants have mechanical properties! This niche area investigates how plant cells and structures withstand and adapt to mechanical forces. It’s useful in agriculture and understanding plant growth patterns.
- Example: Analyzing how tree branches distribute weight helps in urban landscaping and botany.
4. Cellular Biomechanics
This microscopic focus area examines mechanical forces at the cellular level. The biomechanical examples here include how cells deform under pressure or how they migrate during healing.
- Applications: Tissue engineering, cancer research, and regenerative medicine.
5. Occupational Biomechanics
This area applies biomechanics to workplace environments. It aims to design tools, workspaces, and processes that minimize the risk of injury and enhance productivity.
- Example: Redesigning an assembly line to reduce repetitive strain injuries among workers.
Principles of Biomechanics
To truly grasp the meaning of biomechanics, one must understand the core scientific principles that form its foundation.
1. Newton’s Laws of Motion
- First Law (Inertia): A body remains at rest or in uniform motion unless acted upon by an external force.
- Second Law (Force): Force = mass × acceleration. This helps calculate muscle forces during movement.
- Third Law (Action-Reaction): For every action, there's an equal and opposite reaction—key in understanding gait and ground reaction forces.
2. Levers in the Human Body
Our body acts like a system of levers. Muscles and bones work together to create torque and enable movement. There are three classes of levers used for different mechanical advantages.
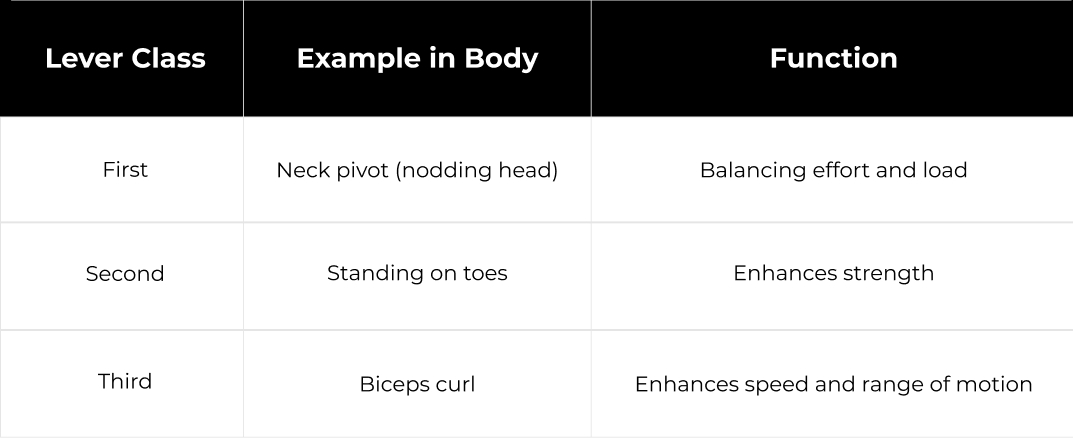
3. Torque, Force Vectors, and Equilibrium
- Torque: Rotational force around a joint. Crucial in movements like throwing or kicking.
- Force Vectors: Used to represent the direction and magnitude of forces.
- Equilibrium: A balanced state where no net force acts on a body—essential for posture and stability.
4. Kinetics vs Kinematics
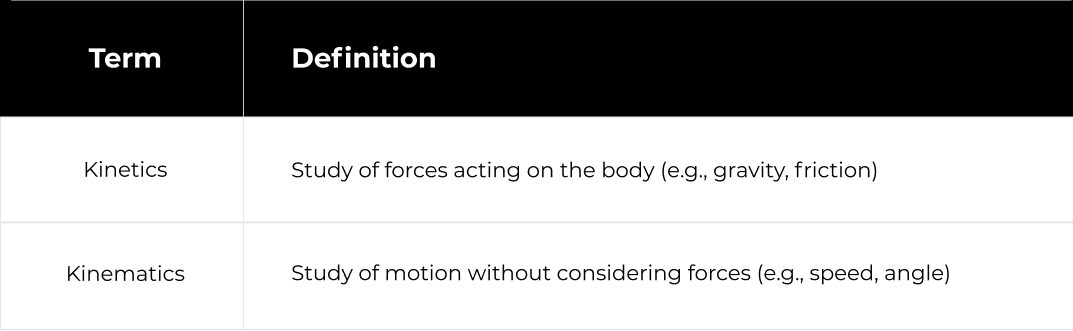
5. Center of Mass and Base of Support
- Center of Mass: The point where a body’s mass is considered concentrated. It shifts based on posture and movement.
- Base of Support: The area beneath a body that supports its weight. A wider base enhances stability and is vital in sports and rehabilitation.
Applications of Biomechanics
1. Sports Science and Athletics
Biomechanics is integral to enhancing athletic performance. Through movement analysis and force assessments, sports scientists fine-tune training programs to suit each athlete's physical makeup.
- Performance Enhancement: By analyzing stride length, jump height, or joint rotation, coaches and biomechanists help athletes improve efficiency and reduce unnecessary movements.
- Injury Prevention: Detailed biomechanical studies can identify improper techniques that may lead to injuries. For example, improper knee alignment in runners can be corrected using gait analysis.
- Equipment Optimization: Customizing gear like shoes, racquets, or helmets using biomechanical insights helps improve performance while reducing the risk of injuries.
2. Medicine and Rehabilitation
One of the most impactful uses of biomechanics is in the medical field.
- Physical Therapy and Orthotics: Therapists use biomechanical examples such as gait correction or limb support design to aid recovery.
- Surgical Planning and Joint Replacements: Orthopedic surgeons rely on biomechanical modeling to simulate joint behavior and ensure optimal implant placement.
3. Ergonomics and Workplace Safety
The study of biomechanics is pivotal in industrial and corporate settings.
- Tool and Workspace Design: Ergonomic designs derived from biomechanics, meaning they ensure comfortable postures and reduce physical strain.
- Repetitive Strain Injury Reduction: Biomech assessments can analyze repetitive actions, especially in factory jobs, can be analyzed for improvement through biomech assessments.
4. Robotics and Prosthetics
In this tech-forward era, biomechanical modeling is being used to enhance robotic efficiency and prosthetic comfort.
- Prosthetic Limbs: Advanced biomechanic simulations ensure that artificial limbs mimic natural movement patterns.
- Human-Robot Interaction: By using biomechanical principles, robots can better understand and mirror human gestures.
5. Forensics and Accident Reconstruction
Biomechanics helps determine the cause and nature of injuries in accident scenarios.
- Motion Analysis: Experts recreate car crashes or falls using motion tracking and biomechanical models to evaluate the sequence of events.
6. Animation and Game Design
Beyond healthcare and sports, biomechanics enhances realism in digital entertainment.
- Realistic Motion Rendering: Characters in films or games move more naturally when designed using real human movement data.
- Motion Capture: Biomechanical tools like markers and sensors are used to translate real-world actions into virtual environments.
Examples of Biomechanics
Let’s look at some biomechanical examples that you’ve likely encountered in everyday life:
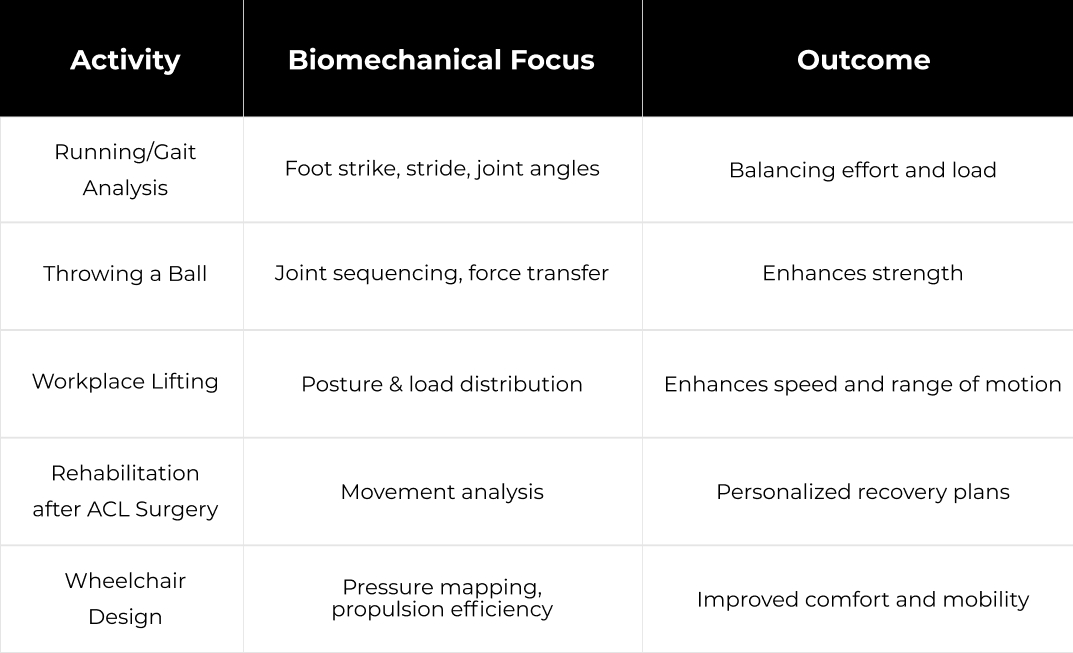
Tools and Techniques Used in Biomechanics
Modern biomechanists rely on a suite of cutting-edge technologies to explore the intricacies of human motion and mechanical function. These tools help decode the complexity of how muscles, bones, and joints interact under various conditions.
Motion Capture Systems
Perhaps the most recognizable tool in the biomechanics lab, motion capture systems are used extensively in sports science, clinical diagnostics, and even game design. These systems use high-speed cameras and reflective markers placed on key anatomical points to capture 3D movement. The collected data allows researchers to study biomechanical patterns like gait, posture, or the kinematics of a golf swing. This is also a staple in animated films and video games for rendering realistic human movement.
Force Platforms
To understand how the body interacts with the ground, force platforms come into play. These sensitive devices measure ground reaction forces—the push-back force that the ground exerts on the body during activities like walking, jumping, or running. Force data, when synchronized with motion capture, provides insights into balance, propulsion, and joint stress, which are crucial for designing better footwear or prosthetics.
Electromyography (EMG)
Electromyography (EMG) reveals what’s happening inside the muscles. By recording the electrical activity of muscles, EMG helps understand how muscles activate and coordinate during movement. It's particularly useful in rehabilitation settings to monitor muscle recovery and optimize physical therapy programs. Surface EMG is commonly used, while fine-wire EMG offers deeper insights but is more invasive.
Computer Modeling and Simulations
Computer simulations are at the heart of predictive biomechanical studies. These digital models replicate bodily structures and simulate real-life conditions, from joint replacements to surgical interventions. This technology allows biomechanists to visualize stress points, predict injury risks, and even optimize performance for athletes without exposing them to real-world strain.
Wearable Sensors
In recent years, wearable sensors have revolutionized biomechanics by bringing it out of the lab and into the real world. These devices—ranging from smart insoles and gyroscopes to accelerometers and posture monitors—track real-time movement data during everyday activities. This has enabled personalized healthcare, remote rehab programs, and ergonomic assessments in work environments.
Video Analysis Software
Sometimes, a simple camera paired with advanced video analysis software can yield powerful biomechanical insights. This software allows frame-by-frame breakdowns of motion, calculation of joint angles, and assessment of movement efficiency. Coaches use it to correct athlete posture; therapists use it to assess recovery progress.

Challenges and Limitations of Biomechanics
While biomechanics offers groundbreaking solutions across diverse fields, it is not without its hurdles. The study of human motion presents several scientific, ethical, and practical challenges.
Human Variability
One of the fundamental limitations in biomechanics is the immense variability in human anatomy and movement. No two individuals move exactly alike. Factors like age, gender, muscle tone, and injury history can influence motion patterns, making it difficult to standardize results or apply one-size-fits-all models. This variability often demands large datasets and personalized approaches.
Ethical Constraints
To gather meaningful biomechanical data, sometimes invasive methods may be proposed—such as inserting sensors into muscles or performing tests that stress joints. However, ethical considerations, especially in clinical populations or children, limit the extent of experimentation. Researchers must always balance scientific goals with participant well-being.
Complexity of Biological Systems
The human body is a marvel of complexity. Muscles, bones, ligaments, and nerves work together in intricate networks. Replicating or simulating these systems accurately is a significant challenge. Small variations in joint motion or tissue elasticity can lead to vastly different outcomes, making accurate modeling in biomechanica (the Spanish equivalent of biomechanics) a demanding task.
High Costs
High-quality biomech equipment such as motion capture labs, EMG devices, or high-speed cameras are not cheap. The cost of equipment and analysis tools remains a barrier for smaller clinics or research institutions. Additionally, trained professionals are needed to operate and interpret the data, adding to the expense.
Future Trends in Biomechanics
As technology continues to advance, biomechanics is undergoing a transformation—becoming more personalized, intelligent, and immersive. These trends are pushing the boundaries of what’s possible in the field.
Integration with AI and Machine Learning
One of the most exciting developments is the integration of artificial intelligence (AI) into biomechanical analysis. AI algorithms can process vast amounts of movement data to recognize patterns, predict injuries, and suggest interventions. This is especially helpful in sports and rehabilitation, where machine learning models can personalize training plans or detect subtle movement errors early.
Personalized Biomechanics Using Wearables
Personalized biomechanics is becoming a reality thanks to smart wearables. Devices like smart insoles, wristbands, or back posture correctors gather live data and offer feedback. This helps users make real-time corrections, improving both safety and performance. In physical therapy, wearables now enable remote sessions, where therapists can monitor a patient's movement patterns from afar.
Bioinspired Robotics
Bioinspired robotics refers to the design of robots that mimic human movement. These systems learn from biomechanical examples of how we walk, grip, or bend. Applications range from robotic limbs for amputees to exoskeletons for warehouse workers or stroke patients. These robots are not only stronger but also more natural in motion—thanks to biomechanical modeling.
Virtual and Augmented Reality
Virtual and augmented reality (VR/AR) is no longer just for gaming. In biomechanics, VR environments are used for motor skill training, sports simulations, and injury rehab. Augmented reality can overlay real-time movement data over the user’s body, offering instant biomechanical feedback. It’s also used in design testing—for example, simulating how a wheelchair might move across different terrains.
Final Thought
In essence, biomechanics is an interdisciplinary marvel that touches nearly every aspect of human life—from the shoes we wear to the way we rehabilitate after surgery. As you walk, run, lift, or even sit, your body is constantly engaging in biomechanical processes. With the integration of AI, smart wearables, and virtual reality, the future holds even more exciting possibilities.
By appreciating the biomechanical meaning behind everyday movements and innovations, we become more aware of the delicate balance between biology and mechanics that governs our world. So next time you see an athlete sprint, or a person using a robotic arm, remember—it’s all biomechanics in action.
FAQs About Biomechanics
- What is the difference between biomechanics and kinesiology?
While biomechanics focuses on the mechanical aspects, kinesiology is the broader study of movement, including physiological and psychological components.
- Is biomechanics only related to sports?
Not at all. Biomechanics is applied in medicine, robotics, workplace safety, and more.
- Can biomechanics help prevent injuries?
Yes, by analyzing movements, it helps correct techniques and avoid strain.
- How is biomechanics used in everyday life?
From the design of ergonomic chairs to smart running shoes, biomechanics shapes daily tools and habits.
- What qualifications do you need to become a biomechanist?
A degree in biomechanics, kinesiology, physical therapy, or biomedical engineering is often required.
- How is biomechanics applied in physical therapy?
Therapists use biomechanical assessments to design tailored recovery plans based on muscle and joint function.
- What are common careers in biomechanics?
Biomechanists work in sports science, research labs, prosthetics companies, gaming studios, and more.